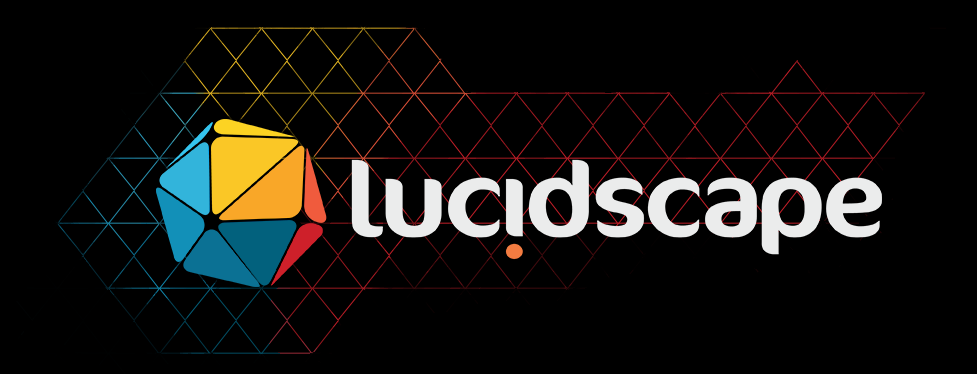
Lucidscape Inc. is a research and development company that explores the intersection of VR/AR, real-time simulations and artificial intelligence.
We are currently working on a neuro-symbolic approach to computer vision that will give autonomous machines richer internal representations of reality.